Introduction
Every good gardener knows that the key to healthy plants is a fertile soil. Plants get water and nutrients from soil and it is the inherent characteristics of the soil in combination with environmental factors that determine soil fertility. Soils are complex and dynamic ecosystems with communities of organisms. Like all ecosystems they have a food web that may include bacteria, fungi, algae, protists, insects, worms, plant roots and burrowing animals. Soils also carry out essential ecosystem functions like water storage and filtration and, perhaps most importantly, decomposition.
Decomposition in soils is a key ecosystem function that in part determines the productivity and health of the plants growing there. Decomposers feed on dead organic matter and in the process break it down into its simplest components: carbon dioxide, water and nutrients (organic matter consists of material or molecules produced by living organisms). The process of decomposition releases large quantities of essential nutrients to the soil solution, thereby making them available to plant roots. In northern hardwood forests, for example, about 85% of a tree’s nitrogen comes from decomposition (Bormann and Likens 1979). Thus, if decomposition of a forest is impaired by drought, acid rain or some other stress, the vegetation may experience nutrient deficiencies.
Students looking for snails at the end of the experiment.
Photograph by T.W. Stewart.
Figure 1. Box and arrow diagram of the terrestrial carbon cycle. |
Decomposition is also important because it is part of the global carbon cycle. The carbon cycle is the cyclical movement of carbon atoms from the atmosphere to the biosphere/lithosphere and back to the atmosphere (Figure 1). In the atmosphere, carbon is in the form of carbon dioxide gas. Through the process of photosynthesis, some of that carbon is converted into organic carbon which makes up organic matter or biomass. Plants and animals perform cellular respiration and convert a small percentage of that organic carbon back to CO2.
A larger portion of that organic carbon in plants is transferred to the soil when plants shed their leaves or when they die. Decomposers then begin their work of breaking down the organic matter. Some of the organic carbon in the organic matter is converted into CO2 which is released into the soil pore spaces leading to relatively high concentrations of CO2 compared to the atmosphere. This difference in concentration causes CO2 to diffuse from the soil to the atmosphere. This movement or flux of CO2 is known as CO2 emission (Figure 1).
Decomposition is not the only source of CO2 in soil. In a forest or grassland ecosystem, plant roots are abundant in the soil and root cells perform cellular respiration, metabolizing carbohydrates that are sent down from the leaves. This CO2 is released to the soil and can be responsible for anywhere between 0 and 60% of a soil’s CO2 emission. Note that CO2 emission is the movement of CO2 from soil to the atmosphere, whereas decomposition and root respiration are processes that produce CO2 in the soil (Figure 2).
Release of CO2 from soils has global implications because it occurs in ecosystems worldwide and its magnitude is such that it contributes significantly to the greenhouse effect. The greenhouse effect is a natural property of our atmosphere in which greenhouse gases prevent the transfer of heat from the earth’s surface to outer space, thereby warming the atmosphere. Since the industrial revolution human activity (e.g., fossil fuel combustion and deforestation) has led to global increases in the concentrations of greenhouse gases (such as CO2) in our atmosphere. This rapid increase will likely lead to a cascade of environmental impacts such as global warming, sea level rise, alteration of precipitation patterns, and increased storm severity (IPCC 2007).
Figure 2. Flow diagram showing the pathway from organic carbon and roots in soil to atmospheric CO2. Boxes represent amounts of carbon (mass) and arrows represent fluxes (mass per unit area per unit time). The italicized terms indicate environmental factors that control the fluxes.
A great deal of research money and effort has been invested in studies of soil CO2 emission in recent years because of the potential impacts of this process on the greenhouse effect (Schlesinger and Andrews 2000). The amount of organic carbon stored in soils worldwide is about 1600 gigatons (Gt) compared to 750 Gt in the atmosphere mostly in the form of CO2 (Rustad et al. 2000). Thus, if soil respiration increased slightly so that just 10% of the soil carbon pool was converted to CO2, atmospheric CO2 concentrations in the atmosphere could increase by one-fifth!
Several environmental factors control the rates of decomposition and root respiration and therefore, the rate of CO2 emission from soils. Since decomposition is an enzyme-mediated biological process carried out by bacteria and fungi, it is very sensitive to temperature. In most soils, the decomposition rate peaks at about 25°C and declines as temperature varies from this maximum. Soil moisture also affects the activity of microorganisms. Very dry or very wet (flooded) conditions tend to reduce decomposition rates (Hanson et al. 1993). A history of acid deposition can also lower the pH of soils thereby inhibiting decomposers.
Respiration rates will also depend on how fast CO2 molecules can diffuse to the soil surface. Diffusion will be affected by soil moisture (how much of the pore space is filled with water) and soil texture (the size distribution of soil particles). Thus, it is likely that soil temperature, moisture, pH, density and texture will all influence soil respiration rates. In this exercise, you will investigate the effects of these (and perhaps other) environmental factors on CO2 emission (Figure 2).
One of the most common methods for measuring soil respiration, the soda-lime method, is remarkably easy and does not require expensive equipment. As a result scientists all over the world have employed it (Grogan 1998). Soda lime is a variable mixture of sodium hydroxide (NaOH) and calcium hydroxide (Ca(OH)2) in granular form. It’s commonly used in laboratories as a desiccant because it readily absorbs water vapor from the air. Because of its alkaline properties soda lime also removes carbon dioxide very efficiently from the atmosphere according to these reactions:
2NaOH (s) + CO2 (g) « Na2CO3 (s) + H2O (ads) [1]
Ca(OH)2 (s) + CO2 (g) « CaCO3 (s) + H2O (ads) [2]
Note that for every molecule of CO2 adsorbed, a molecule of water is created. These water molecules remain temporarily adsorbed (ads) to the soda lime but can be evaporated off at boiling temperatures.
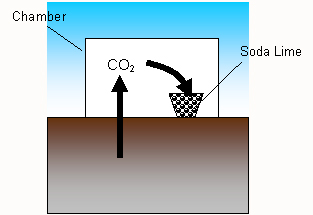
Figure 3. Schematic diagram of the soil respiration chambers. CO2 diffuses from the soil into the chamber air space. It is then absorbed by the soda lime.
The soda lime method involves placing a pre-weighed, open dish of soda lime on the ground and covering it with a chamber of known diameter (Figure 3). As the soil CO2 diffuses into the chamber it is quickly absorbed by the soda lime (along with water vapor). After 24 hours, the chamber is removed and the soda lime is dried at 105°C to evaporate the water and then weighed. The increase in mass of the soda lime is attributable to CO2 (Edwards 1982, as modified by Grogan 1998).
Materials and Methods
Study Site(s): With your Instructor, choose appropriate study sites that are relatively flat and are not extremely stony. You need to be able to place an 18 cm (7.1 in) diameter chamber on the ground where there are no living plants and no large stones. Depending on your experimental question you may want two contrasting sites like conifer site vs. hardwood site, north slope vs. south slope, or dry vs. wet.
Overview of Data Collection and Analysis Methods:
1 to 2 Days Before Lab Session 1:
- Label glass jars (40- to 100-mL glass jar with screw top) with a piece of tape and permanent marker. Add approximately 8 grams of soda lime to each jar. Place the jars with soda lime in an oven at 105°C for at least 24 hours to evaporate the water from the granules. You will need 8 - 10 jars per group plus one extra that the whole class can use for the blank.
Lab Session 1:
- Remove jars from the oven (use gloves or tongs!) and place in a desiccator to cool for 2-5 minutes. Remove jars from desiccator one at a time, weigh to the nearest milligram (0.001 g) or tenth-milligram (0.0001g) and cover immediately. Record the mass as the initial mass in Table 1.
- Take the jars, chambers, thermometers and sampling equipment and go out to your field site. Take a few minutes to note the variations in microclimate and microtopography within the forest.
- In small groups design your experiment. You will be comparing the rate of soil CO2 emission of two sites with different microclimates and/or soil characteristics. As a group, decide on the sites or the microclimates you would like to compare. Here are some suggestions but you are encouraged to think of your own:
Conifer site vs. hardwood site
Sun vs. shade
Ridgetop vs. valley bottom
With leaf layer vs. without leaf layer (i.e., the layer of dead leaves on the soil surface is removed)
- As a group write out your Experimental Design according to the handout, Experimental Design Requirements. Show it to your Instructor for approval before proceeding. As homework type up your answers to the questions on the handout.
- Place a chamber upside down on a relatively flat area of the soil. The entire rim of the chamber must be inserted at least 1 cm into the soil so as to minimize gas exchange with the atmosphere. So, carefully remove twigs and small rocks that lie under the rim without disturbing the leaves and soil surface under the chamber. Remove any green plants by pinching or cutting them at soil level. It is essential that the soil be disturbed as little as possible!
- Slowly and carefully push down while rotating the chamber back and forth to force the edges about 1 – 2 cm into the soil surface. If there are subsurface roots or rocks in the way, you may need to move to another location. The key here is to get a good seal all along the edge of the chamber so there are no gaps.
- Obtain a jar containing soda lime. Remove the cap and place the jar under the chamber so that it rests on the soil surface. Make sure it is not likely to tip over.
- Replace the chamber and place a weight on it (like a fist-sized rock or a thick branch) to maintain pressure and keep it from blowing away or tipping over.
- Record the number of the soda lime jar and the number and location of the chamber. Repeat these steps for each of the chambers at each site.
- At one of the sites used by the class, place an opened jar of soda lime in an upright chamber and seal the chamber with a lid. This will serve as a blank to document the amount of CO2 absorbed from the air in the chamber and during the opening and closing of the jars. Only one blank is needed for all of the groups.
- Let all chambers incubate for 24 (+ 4) hours. If the ambient daytime air temperature is below 16ºC, then incubate the chambers for 48 (+ 4) hours.
- Before leaving the site quantify the differences in environmental factors between your two sampling sites. You may measure any or all of the following. Your Instructor may have additional parameters for you to measure. Click here for instructions on measuring these variables.
Soil temperature
Soil moisture
Soil pH
1 or 2 Days After Lab Session 1:
- Return to the field site after the designated time has elapsed. Remove the chambers and cap the soda lime jars. Return all materials to the lab. Uncover the soda lime jars and place them in the drying oven at 105ºC.
Lab Session 2:
- Remove the dry soda lime from the oven and place in a desiccator to cool for 5 minutes. Remove jars one at a time from the desiccator, weigh to the nearest milligram (0.001 g) or tenth-milligram (0.0001 g). Record this as the final mass (which includes the mass of the jar) in Table 1.
- Calculate the mg of soil CO2 absorbed by the soda lime in each chamber:
Change in Mass of Blank (g) = Mb = (Final Mass of Blank – Initial Mass of Blank)
Soil CO2 Absorbed (g) = Final Mass – Initial Mass – Mb
- Calculate the CO2 Emission Rate (E) for each chamber:
Ac = Area of ground covered by chamber (m2)
E (g CO2 m-2 d-1) = (Soil CO2 Absorbed * 1.69) / Ac / Days of Incubation
[The 1.69 in the equation above is used to correct for the water molecule that is lost when a molecule of CO2 is adsorbed.]
Click here for a data sheet in EXCEL format.
- Perform a Student’s t-Test on the CO2 Emission Rates to test for significant differences between the two experimental treatments. Click here for a step-by-step procedure.
- With help from the Instructor summarize your environmental variables and create a table in the proper format to present these data.
Homework:
Write a lab report using the proper format. Click here for report guidelines. Your Instructor will assign a due date for the first draft of the report and for the final draft of the report.
Questions for Further Thought and Discussion
- How did your two sampling sites differ in terms of temperature, moisture, pH or other characteristics? Could these differences explain the differences you observed in CO2 emission rate?
- The soil under your chambers probably contained plant roots. How might these plant roots have affected your CO2 emission rates? Explain. Design an experiment using these chambers that would allow you to determine what proportion of the CO2 emitted came from roots and what proportion came from decomposition.
- Explain how decomposition in soils is linked to the greenhouse effect.
- If just 5% of the world’s soil organic carbon pool was decomposed , how many tons of carbon would be released?
- Calculate the average CO2 emission rate and standard deviations for each sample location (or perform a statistical test). Put these values in a table. Then write two to three paragraphs describing and interpreting the results of your experiment.
- The temperature and moisture data you collected represent point-in-time measurements. Do you think the temperatures and soil moisture values are representative of the microclimate during the entire incubation period? What would be a more accurate way to quantify the microclimate during the incubation period?
- Are there other environmental or site factors that you did not measure that could explain the differing rates of CO2 emission between your sampling locations? Explain how they would affect the emission rate.
- CO2 Emission varies with geographic location and with season. Conduct a literature search for soil CO2 emission values from around the world. Try to find some from your area. Some key words that will aid you in your search are: soil respiration, soil CO2, soil carbon, carbon emissions, CO2 emissions, soda lime, carbon cycle. What range of values can you find? Where are the values the highest? Where are they the lowest? How does your area compare? [Note: make sure when you compare values from different studies that you convert all the values to the same units!]
- Because decomposition is a temperature-dependent process, it is expected to be affected by global warming. Write down one or two predictions about how decomposition in soil will change and how those changes will affect plants. Then conduct a literature search to find out what the experts are predicting. Were your predictions correct? If not, why not? What other predictions have the experts made? Some search phrases that will aid you in your search are: soil CO2, CO2 emissions, soil respiration, global warming soil carbon, tundra soils, global warming positive feedback, soil respiration temperature, decomposition temperature.
References and Links
- Bormann, F.H. and G.E. Likens. 1979. Pattern and Process in a Forested Ecosystem. Springer-Verlag, New York, NY.
- Buchman, N. 2000. Biotic and abiotic factors controlling soil respiration rates in Picea abies stands. Soil Biology and Biochemistry 32:1625-1635.
- Edwards, N.T. 1982. The use of soda-lime for measuring respiration rates in terrestrial systems. Pedobiologia 23:321-330.
- Grogan, P. 1998. CO2 flux measurement using soda lime: correction for water formed during CO2 adsorption. Ecology 79:1467-1468.
- Hanson, P.J., S.D. Wullschleger, S.A. Bohlman, and D.E. Todd. 1993. Seasonal and topographic patterns of forest floor CO2 efflux from an upland oak forest. Tree Physiology 13:1-15.
- Hogberg, P., A. Nordgren, N. Buchmann, A.F. Taylor, A. Ekblad, M.N. Hogberg, G. Nyberg, M. Ottosson-Lofvenius, D.J. Read. 2001. Large scale forest girdling shows that current photosynthesis drives soil respiration. Nature 411:789-92.
- IPCC, 2007: Climate Change 2007: The Physical Science Basis. Contribution of Working Group I to the Fourth Assessment Report of the Intergovernmental Panel on Climate Change [Solomon, S., D. Qin, M. Manning, Z. Chen, M. Marquis, K.B. Averyt, M. Tignor and H.L. Miller (eds.)]. Cambridge University Press, Cambridge, United Kingdom and New York, NY, USA, 996 pp.
- Raich, J.W., and W.H. Schlesinger. 1992. The global carbon dioxide flux in soil respiration and its relationship to vegetation and climate. Tellus 44B:81-99.
- Rustad, L.E., T.G. Huntington, R.D. Boone. 2000. Controls on soil respiration: Implications for climate change. Biogeochemistry 48:1-6.
- Schlesinger, W.H., and J.A. Andrews. 2000. Soil respiration and the global carbon cycle. Biogeochemistry. 48:7-20.
- Simmons, J.A., I.J. Fernandez, R.D. Briggs and M.D. Delaney. 1996. Forest floor carbon pools and fluxes along a regional climate gradient in Maine, USA. Forest Ecology and Management 84:81-95.
- Toland, D.E., and D.R. Zak. 1994. Seasonal patterns of soil respiration in intact and clear-cut northern hardwood forests. Canadian Journal of Forest Research 24:1711-1716.
Useful Web Sites
- Caprette, D.R. 2005. Student’s t-Test (for independent samples). (http://www.ruf.rice.edu/~bioslabs/tools/stats/ttest.html, accessed 4 December 2007)
- Davidson, E. 2006. Soil respiration, temperature and moisture. (http://harvardforest.fas.harvard.edu/data/p00/hf006/hf006.html, accessed 12 December 2007)
- Dolphin, W.R. 1997. Writing Lab Reports and Scientific Papers. (http://www.mhhe.com/biosci/genbio/maderinquiry/writing.html, accessed 13 December 2007)
- Environmental Literacy Council. 2006. Soil and the Carbon Cycle. (http://www.enviroliteracy.org/article.php/700.html, accessed 4 December 2007)
- Harmon, M. 2003. LIDET: Long-term intersite decomposition experiment team. (http://www.fsl.orst.edu/lter/research/intersite/lidet.htm, accessed 13 December 2007)
- Rice, C.W. 2005 (posting date). What is the carbon cycle? (http://soilcarboncenter.k-state.edu/carbcycle.html, accessed 4 December 2007)
- Roche, J. 2007. How to write a lab report.
(http://inpp.ohiou.edu/~roche/371_fall07/how_to_write_a_lab_report.pdf, accessed 10 December 2007)
- Trochim, W.M.K. 2006. The t-test. (http://www.socialresearchmethods.net/kb/stat_t.php, accessed 13 December 2007)
United States Geological Survey. 2006. Assessing carbon stocks in soil. (http://edcintl.cr.usgs.gov/carbon_cycle/carbonstocks.html, accessed 11 December 2007)
- Woods Hole Research Center. 2005. Soil Respiration. (http://www.whrc.org/new_england/Howland_Forest/soil_respiration.htm, accessed 5 December 2007)
Tools for Assessment of Student Learning Outcomes
Assessment
You will be assessed on two aspects of this project - the experimental design and the written lab report. The experimental design will be used to assess your ability to use the scientific method appropriately to answer a question. The lab report will be used to test your comprehension of the principles behind soil respiration and your ability to communicate in writing in proper scientific format.
Experimental Design Guidelines
Download Experiment Design Guidelines document (DOC)
Lab Report Guidelines
See Lab Report Guidelines document (DOC)
Rubrics
Download Experimental Design Rubric (DOC)
Download Lab Report Prime Trait Assessment (EXCEL file (XLS) and WORD file (DOC))
Sample Exam Questions
Q. The process that converts atmospheric CO2 into organic C in plants is_________.
A. Photosynthesis
Q. If global warming were to lead to warmer soil temperatures and therefore faster decomposition worldwide, what would you expect to happen to the levels of CO2 in the atmosphere (all else being equal)? Explain.
A. Faster decomposition would lead to greater CO2 emission rates which would lead to an increase in atmospheric CO2 concentration.
Q. Acid deposition tends to inhibit soil microbial populations and lead to slower decomposition. What effect, if any, will this have on the vegetation? Explain.
A. Plants obtain most of their nutrients from the decomposition process. If decomposition is slowed, plants may become nutrient deficient or their growth will be slowed .